

Original Article - Year 2012 - Volume 27 -
Effects of different liposuction techniques on the isolation of mesenchymal stem cells
Efeitos de diferentes pressões de aspiração do tecido adiposo na obtenção de células-tronco mesenquimais
ABSTRACT
BACKGROUND: In recent years, fat grafts have become useful in plastic surgery. They are mainly used to fill soft tissues, refine breast reconstructions, and for volumetric facial rejuvenation. They are also a rich source of mesenchymal stem cells (i.e., adipose-derived stem cells [ADSCs]), which directly influence fat graft survival. Since ADSCs play an important role in angiogenesis and adipogenic differentiation, it is essential to optimize their isolation. Therefore, in this study, we evaluated and compared 2 procedures used to isolate viable cells from the stromal vascular fraction of abdominal adipose tissue and assess the expressions of surface markers.
METHODS: We examined 9 female subjects who were scheduled to undergo liposuction. The adipose tissue was isolated from the abdominal infraumbilical region. Fat (20 mL) was collected from the right side by using a cannula attached to a syringe; the plunger was pulled back every 2 cm3 to create low-pressure suction (manual group). The same procedure was repeated on the left side, but the cannula was attached to a sterile and intermediate collector coupled to a vacuum pump that provided a constant negative pressure of 350 mmHg (pump group). The samples were centrifuged, and the adipocytes of the intermediate layer were counted, cultured, and immunophenotyped.
RESULTS: The isolation of abdominal adipocytes with a pump providing a negative pressure of 350 mmHg yielded a higher concentration of cells in the stromal vascular fraction than that obtained using 10-mL syringes and low-pressure suction, although the difference was not significant.
CONCLUSIONS: A negative pressure of 350 mmHg may be safely applied to isolate ADSCs. The cell yield did not indicate any statistically significant difference between the techniques.
Keywords: Immunophenotyping. Stem cells. Mesenchymal stem cell transplantation. Subcutaneous fat, abdominal. Regenerative medicine.
RESUMO
INTRODUÇÃO: O enxerto de gordura nos últimos anos voltou a ter destaque como aliado dos cirurgiões plásticos no preenchimento de partes moles, no rejuvenescimento facial volumétrico, nos refinamentos de reconstruções mamárias e por ser rica fonte de células-tronco de comportamento mesenquimal (células-tronco adipoderivadas). Considerando que essas células têm importante papel na angiogênese e na diferenciação adipogênica, com impacto direto na sobrevivência dos enxertos de gordura, determinar parâmetros que otimizem a sua obtenção é imperativo. Nesse contexto, o objetivo deste trabalho é avaliar e comparar dois métodos de obtenção do tecido adiposo da região abdominal quanto ao número de células viáveis presentes na fração vásculo-estromal e analisar a expressão de marcadores de superfície.
MÉTODO: Foram selecionadas 9 pacientes do sexo feminino submetidas a lipoaspiração. O tecido adiposo foi obtido da região abdominal infraumbilical. Da metade direita foram coletados 20 ml de gordura, empregando-se cânula acoplada a uma seringa, cujo êmbolo foi tracionado de 2 cc em 2 cc, gerando baixas pressões de aspiração (grupo manual). O mesmo processo foi repetido na metade esquerda, entretanto a cânula estava acoplada a um coletor intermediário estéril e esse a uma máquina de vácuo sob pressão negativa constante de 350 mmHg (grupo a vácuo). As amostras foram centrifugadas e a gordura da camada intermediária dos dois grupos foi submetida a contagem celular, estabelecimento de culturas e posterior imunofenotipagem.
RESULTADOS: Este estudo demonstrou que, apesar de não haver diferença estatisticamente significativa, a obtenção da gordura da região abdominal empregando-se lipoaspirador com pressão negativa de 350 mmHg proporcionou maior número de células presentes na fração vásculo-estromal quando comparado à obtenção por meio de seringas de 10 ml, com baixas pressões de aspiração.
CONCLUSÕES: O emprego de pressão negativa de 350 mmHg é seguro para a obtenção das células-tronco adipoderivadas e o rendimento celular entre os dois grupos não apresentou diferença estatisticamente significativa.
Palavras-chave: Lipectomia. Imunofenotipagem. Células-tronco. Transplante de células-tronco mesenquimais. Gordura subcutânea abdominal. Medicina regenerativa.
Since the advent of fat grafts in 1893, this technique has been used as an important procedure to fill soft tissues1. Fat grafts were originally isolated as small or large fragments1,2; subsequently, they could be isolated as smaller fat globules due to the introduction of liposuction3. Coleman4 developed a technique that systematized the isolation, preparation, and transfer of adipose tissue in the recipient bed to improve the graft integration of differentiated adipocytes and provide long-lasting clinical results.
The physiology of fat graft integration involves an initial phase of plasmatic imbibition, angiogenesis, survival of mature adipocytes, and adipogenic differentiation of the graft and recipient bed precursors5. After the discovery of pre-adipocytes in 1926 1, several experimental studies demonstrated the ability of these precursors to develop into differentiated and functional adipose tissue6-8. Zuk et al.9 isolated cells in the stromal vascular fraction of adipose tissue that could undergo pluripotent differentiation in a similar manner to the mesenchymal stem cells of the bone marrow. These cells were termed as adipose-derived stem cells (ADSCs).
The adipose tissue is used as a rich source of mesenchymal stem cells for tissue engineering and regeneration. Therefore, optimizing the procedures for isolating and preparing ADSCs is necessary to achieve the highest number of cells per gram of suctioned adipose tissue. In this study, we evaluate and compare the 2 current procedures used to isolate viable cells from the stromal vascular fraction of abdominal adipose tissue, and assess the expressions of surface markers.
METHODS
We examined 9 healthy female subjects who were scheduled to undergo cosmetic liposuction under general anesthesia. All subjects signed an informed consent form approved by the Research Ethics Committee of the Institute of Biosciences of the University of São Paulo.
Adipose tissue was harvested from the infraumbilical abdominal region. Twenty milliliters of fat was collected from the right side using a cannula (2.5 mm in diameter and 15 cm in length) coupled to a 10 cm3 screw-thread type syringe. The plunger of the syringe was pulled back every 2 cm3 to generate low-pressure suction. This group was called the manual group.
In addition, 20 mL fat was collected from the left side, using a cannula (3 mm in diameter and 25 cm long) coupled to an intermediate sterile collector attached to a pump providing a negative constant pressure of 350 mmHg. This group was called the pump group.
The samples were appropriately labeled and then centrifuged for 3 minutes at 700 × g. Three separate layers were obtained after centrifugation, and the upper and lower layers were discarded. The fat obtained by isolating the intermediate layer in the 2 sample groups was immediately sent to the Laboratory of Genetics of the Institute of Bioscience of the University of São Paulo for analysis. Cells present in the samples were subsequently counted, cultured, and immunophenotyped.
Fat samples were subjected to enzymatic digestion with 0.5 mg/mL collagenase type Ia (Sigma Aldrich) and maintained for 30 minutes in a water bath at 37ºC. The more viscous liquid phase was collected, and the collagenase was inactivated in Hank's balanced salt solution (Gibco, Invitrogen). The suspension was centrifuged for 5 minutes at 500 × g to precipitate cells; the supernatant was discarded.
The cell pellets were resuspended, and each sample was divided into 4 aliquots. One aliquot was stained with 0.05% Trypan blue for automated cell counting (Countess Automated Cell Counter, Invitrogen); the final concentration was determined by counting the cells present in each cubic centimeter of digested fat. Another aliquot was cryopreserved for further analysis, when required.
The remaining aliquots were seeded in duplicate in 75-cm2 plastic flasks in DMEM/F12 medium supplemented with 1% MEM non-essential amino acid solution, 1% streptomycin, 1% penicillin (Gibco, Invitrogen), and 10% fetal bovine serum (Hyclone). The flasks were maintained in the incubator at 37ºC with 5% CO2. The culture medium was changed every 3 days to obtain 85% cell confluence at passage zero (P0).
Thereafter, adherent cells were washed with buffered saline solution and detached with trypsin (0.125% trypsin, 0.02% EDTA in PBS). Small aliquots of 5 × 103 cells each were prepared and stained with monoclonal IgG antibodies against CD29, CD 31, CD34, CD44, CD45, CD73, CD90, and CD105 membrane proteins. Flow cytometry was performed with the Guava EasyCyte, and the results were analyzed with the Guava ExpressPlus program (Guava Technologies Hayward).
Paired Student's t-tests were used to compare the number of cells obtained in the manual and pump groups. The level of significance was set at P < 0.05.
RESULTS
The number of cells isolated from the fat stromal vascular fraction of the infraumbilical region varied greatly among the 9 female subjects; the average age of the subjects was 46.7 years (range, 22-76 years), and average body mass index (BMI) was 24.9 kg/m2 (range, 22-29.6 kg/m2) (Table 1).
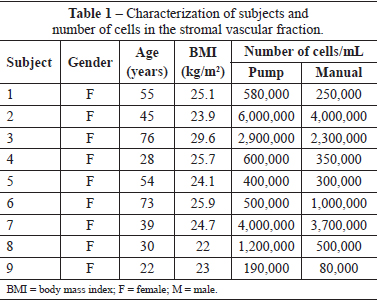
The average number of cells isolated using the manual and pump techniques were 1.35 × 106 and 1.77 × 106 cells, respectively.
Although the pump group tended to have more cells than the manual group (Figure 1), the difference was not significant (P = 0.094).
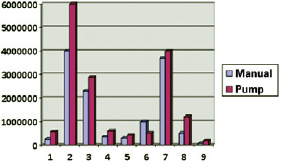
Figure 1 - Cell yield in the manual and pump groups.
The immunophenotyping performed in P0 cells showed no expression and very low expression of endothelial (CD31-) and hematopoietic lineage markers (CD34+ and CD45+), respectively (Figure 2). However, the markers present in the mesenchymal cell population (i.e., CD29+, CD44+, CD 73+, CD90+, and CD105+) appear to be highly expressed in cells isolated from the stromal vascular fraction of the abdominal adipose tissue harvested using both techniques (Figure 3).
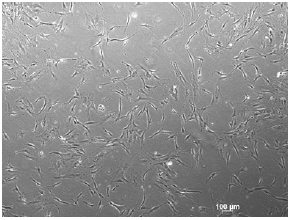
Figure 2 - Cells isolated from the stromal vascular fraction of adipose tissue were seeded in flasks and cultured in growth medium until confluence. Cells exhibited an elongated shape with several dendritic extensions.
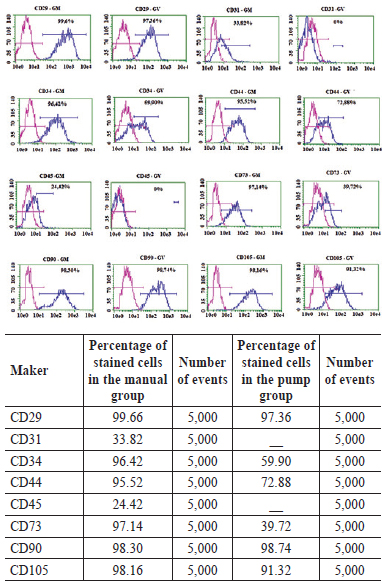
Figure 3 - Percentages of stained cells with different markers and numbers of events in both the manual and pump groups. In the above graphs, the magenta lines indicate the control, whereas the blue lines indicate the expressions of surface markers, which was similar in the manual and pump groups. MANUAL, manual group; PUMP, pump group.
DISCUSSION
A long-term review of fat grafts reveals the high variability of results obtained with this procedure. However, more satisfactory results were achieved by authors who developed procedures aimed to manipulate and harm the least possible number of adipocytes during isolation, preparation, and grafting10,11. The last phase requires appropriate cellular nutrition and respiration, achieved by plasmatic imbibition, which leads to neoangiogenesis5. The graft requires a higher concentration of adipocyte precursors of the stromal vascular fraction because of their crucial role in neoangiogenesis, adipogenic differentiation, and consequent long-term volumetric maintenance of the graft12,13.
The number of nucleated cells assessed by cell counting was higher in the stromal vascular fraction of the pump group, although the difference was not significant. Although the Trypan blue assay is not considered a direct cell viability assay since it does not take into consideration cellular metabolism, it was used to stain cells of the stromal vascular fraction of the adipose tissue, which include ADSCs, macro-phages, pericytes, endothelial and hematopoietic stem cells, pre-adipocytes, and fibroblasts among others.
Immunophenotyping revealed that the negative pressure did not alter the expressions of surface markers at P0. Moreover, the results demonstrate that the ability of adipogenic differentiation remained unchanged. In addition, the expression profile of surface markers in P0 cells was similar to that found in the literature14.
Several substances are added to fat grafts to improve adipocyte integration, including interleukin-8 15, platelet-rich plasma16, insulin17, and AMD 3100 18. However, the use of such substances is restricted to experimental biology because of the controversial results obtained with their use. The discovery of pluripotent ADSCs9 and establishment of a simple procedure to isolate these cells led Yoshimura et al.19 to develop fat grafts enriched with ADSCs to treat facial lipodystrophy and hypomastias20 as well as complications due to silicone breast implants21. However, the integration rates of these grafts vary greatly.
Further clarification is required to elucidate the role of precursor adipose cells in the integration and in the nourishment of fat grafts with stromal vascular cells of differentiated adipose tissue. The absence of randomized prospective studies comparing long-term clinical results of fat grafts with and without cell enrichment means that it remains unconfirmed whether both increased ADSC graft concentration and their prior stimulation should be performed to achieve a higher integration rate. However, procedures optimizing the isolation of cells from the stromal vascular fraction are required.
This study supports the findings of Mojallal et al.22 and demonstrates that manual aspiration with 10 cm3 syringes and low vacuum pressure lyse fewer mature adipocytes, although fewer cells are isolated from the stromal vascular fraction. Aguena et al.23 show that isolation of cells from the lower abdomen below the navel yields more nucleated cells in the stromal vascular fraction than that obtained from the flanks. These findings support those of Jurgens et al.24.
Although significant individual variation was present, the cell yield obtained per cubic centimeter fat is concordant with that observed in the literature25. Moreover, the time required to reach 85% cell confluence was the same in both groups even though the formation of colony-forming units (CFU-F) was not assessed in this study. This observation demonstrates that a negative pressure of 350 mmHg does not alter cell growth (i.e., doubling time). Kurita et al.26 show that nucleated cells of the stromal vascular fraction of adipose tissue may be centrifuged at relative forces up to 4200 × g.
Concordant with the results published by Mitchell et al.14, homogenous immunophenotyping at P0 was observed in both the manual and pump groups. Moreover, antibody tests indicated the presence of surface markers of mesenchymal stem cell lineage, proving their ability to undergo adipogenic differentiation.
CONCLUSIONS
The results of the present study show that a negative pressure of 350 mmHg may be safely applied to isolate ADSCs and that the cell yields between the manual and pump techniques are not significantly different. The possibility of using these cells in surgery to enrich fat grafts immediately after their extraction requires that immunophenotyping should be performed in the fresh stromal vascular fraction. However, further studies should be performed to establish the optimal enrichment rate of fat grafts with ADSCs to improve long-term clinical outcomes.
REFERENCES
1. Billings E Jr, May JW Jr. Historical review and present status of free fat graft autotransplantation in plastic and reconstructive surgery. Plast Reconstr Surg. 1989;83(2):368-81.
2. Ellenbogen R. Fat transfer: current use in practice. Clin Plast Surg. 2000;27(4):545-56.
3. Illouz YG. Body contouring by lipolysis: a 5-year experience with over 3000 cases. Plast Reconstr Surg. 1983;72(5):591-7.
4. Coleman SR. Facial recontouring with lipostructure. Clin Plast Surg. 1997;24(2):347-67.
5. Coleman SR. Structural fat grafting: more than a permanent filler. Plast Reconstr Surg. 2006;118(3 suppl.):108S-20S.
6. Hausberger FX. Quantitative studies on the development of autotransplants of immature adipose tissue of rats. Anat Rec. 1955;122(4):507-15.
7. Van RL, Roncari DA. Complete differentiation in vivo of implanted cultured adipocyte precursors from adult rats. Cell Tissue Res. 1982;225(3):557-66.
8. Rodeheffer MS, Birsoy K, Friedman JM. Identification of white adipocyte progenitor cells in vivo. Cell. 2008;135(2):240-9.
9. Zuk PA, Zhu M, Mizuno H, Huang J, Futrell JW, Katz AJ, et al. Multilineage cells from human adipose tissue: implications for cell-based therapies. Tissue Eng. 2001;7(2):221-28.
10. Pu LL, Coleman SR, Cui X, Ferguson RE Jr, Vasconez HC. Autologous fat grafts harvested and refined by the Coleman technique: a comparative study. Plast Reconstr Surg. 2008;122(3):932-7.
11. Ramon Y, Shoshani O, Peled IJ, Gilhar A, Carmi N, Fodor L, et al. Enhancing the take of injected adipose tissue by a simple method for concentrating fat cells. Plast Reconstr Surg. 2005;115(1):197-201.
12. Planat-Bernard V, Silvestre JS, Cousin B, André M, Nibbelink M, Tamarat R, et al. Plasticity of human adipose lineage cells toward endothelial cells: physiological and therapeutic perspectives. Circulation. 2004;109(5):656-63.
13. Rehman J, Traktuev D, Li J, Merfeld-Clauss S, Temm-Grove CJ, Bovenberk JE, et al. Secretion of angiogenic and antiapoptotic factors by human adipose stromal cells. Circulation. 2004;109(10):1292-8.
14. Mitchell JB, McIntosh K, Zvonic S, Garrett S, Floyd ZE, Kloster A, et al. Immunophenotype of human adipose-derived cells: temporal changes in stromal-associated and stem cell-associated markers. Stem Cells. 2006;24:376-85.
15. Shoshani O, Livne E, Armoni M, Shupak A, Berger J, Ramon Y, et al. The effect of interleukin-8 on the viability of injected adipose tissue in nude mice. Plast Reconstr Surg. 2005;115(3):853-9.
16. Salgarello M, Visconti G, Rusciani A. Breast fat grafting with platelet-rich plasma: a comparative clinical study and current state of the art. Plast Reconstr Surg. 2011;127(6):2176-85.
17. Lu F, Li J, Gao J, Ogawa R, Ou C, Yang B, et al. Improvement of the survival of human autologous fat transplantation by using VEGF-transfected adipose-derived stem cells. Plast Reconstr Surg. 2009;124(5):1437-46.
18. Butala P, Sultan SM, Davidson EH, Crawford JL, Szpalski C, Knobel D, et al. Progenitor cell mobilization augments fat graft survival. Plast Reconstr Surg. 2010;126(4):56-7.
19. Yoshimura K, Sato K, Aoi N, Kurita M, Inoue K, Suga H, et al. Cell-assisted lipotransfer for facial lipoatrophy: efficacy of clinical use of adipose-derived stem cells. Dermatol Surg. 2008;34(9):1178-85.
20. Yoshimura K, Sato K, Aoi N, Kurita M, Hirohi T, Harii K. Cell-assisted lipotransfer for cosmetic breast augmentation: supportive use of adipose-derived stem/stromal cells. Aesthetic Plast Surg. 2008;32(1):48-55.
21. Yoshimura K, Asano Y, Aoi N, Kurita M, Oshima Y, Sato K, et al. Progenitor-enriched adipose tissue transplantation as rescue for breast implant complications. Breast J. 2010;16(2):169-75.
22. Mojallal A, Auxenfans C, Lequeux C, Braye F, Damour O. Influence of negative pressure when harvesting adipose tissue on cell yield of the stromal-vascular fraction. Biomed Mater Eng. 2008;18(4-5):193-7.
23. Aguena M, Fanganiello RD, Tissiani LA, Ishiy FA, Atique R, Alonso N, et al. Optimization of parameters for a more efficient use of adipose-derived stem cells in regenerative medicine therapies. Stem Cells Int. 2012;2012:303610.
24. Jurgens WJ, Oedayrajsingh-Varma MJ, Helder MN, Zandiehdoulabi B, Schouten TE, Kuik DJ, et al. Effect of tissue-harvesting site on yield of stem cells derived from adipose tissue: implications for cell-based therapies. Cell Tissue Res. 2008;332(3):415-26.
25. Aust L, Devlin B, Foster SJ, Halvorsen YD, Hicok K, du Laney T, et al. Yield of human adipose-derived adult stem cells from liposuction aspirates. Cytotherapy. 2004;6(1):7-14.
26. Kurita M, Matsumoto D, Shigeura T, Sato K, Gonda K, Harii K, et al. Influences of centrifugation on cells and tissues in liposuction aspirates: optimized centrifugation for lipotransfer and cell isolation. Plast Reconstr Surg. 2008;121(3):1033-41.
1. Plastic surgeon, Doctoral student of the postgraduate program of the Faculdade de Medicina da Universidade de São Paulo (Faculty of Medicine, University of São Paulo) - FMUSP, member of the Sociedade Brasileira de Cirurgia Plástica (Brazilian Society of Plastic Surgery) - SBCP, São Paulo, SP, Brazil.
2. Doctor in biological sciences, Human genome biologist, Instituto de Biociências da Universidade de São Paulo (Institute of Bioscience, University of São Paulo), São Paulo, SP, Brazil.
3. Full professor at the Department of Evolutionary Genetics at the Instituto de Biociências da Universidade de São Paulo (Institute of Bioscience, University of São Paulo), São Paulo, SP, Brazil.
4. Associate professor at FMUSP, plastic surgeon, member of the SBCP, São Paulo, SP, Brazil.
Correspondence to:
Nivaldo Alonso
Rua Afonso Braz, 473 - cj. 65 - Vila Nova Conceição
São Paulo, SP, Brazil - CEP: 04511-010
E-mail: nivalonso@gmail.com
Submitted to SGP (Sistema de Gestão de Publicações/Manager Publications System) of RBCP (Revista Brasileira de Cirurgia Plástica/Brazilian Journal of Plastic Surgery).
Article received: July 15, 2012
Article accepted: October 30, 2012
This study was performed at the Hospital Municipal Carmino Caricchio (Municipal Hospital Carmino Caricchio), Instituto de Biociências and Faculdade de Medicina da Universidade de São Paulo (Institute of Bioscience and Faculty of Medicine, University of São Paulo), São Paulo, SP, Brazil.