

Original Article - Year 2011 - Volume 26 - Issue 3
Role of keratinocytes in wound contraction: an impact assessment using a model of collagen matrix populated with fibroblasts
Papel do queratinócito na contração da ferida: avaliação de impacto usando um modelo de matriz de colágeno povoada por fibroblastos
ABSTRACT
Background: The possible participation of keratinocytes in wound remodeling has been widely studied. This study investigated the impact of keratinocytes in wound contraction. Methods: Murine type I collagen gels populated by human fibroblasts and seeded with human keratinocytes on the surface to form a dermo-epidermal equivalent were used as the study group. Collagen gels populated by only fibroblasts were used as the control group. The criteria for the preparation and storage of gels were similar for both groups. Results: An evident and statistically significant increase in gel contraction was observed in samples populated by keratinocytes compared to the control group. Conclusions: These results suggest that keratinocytes not only modulate fibroblast proliferation but also play an active role in wound contraction per se. Further research on the mechanisms involved in the communication pathways between cells and between cells and the matrix shall be assessed from the perspective of keratinocyte participation in wound healing and pathologic scarring.
Keywords: Keratinocytes. Wound healing. Cell culture techniques. Fibroblasts.
RESUMO
Introdução: A eventual participação de queratinócitos na remodelagem da ferida tem sido estudada há muito tempo. Este trabalho investigou o impacto dos queratinócitos na contração da ferida. Métodos: Foi utilizado gel de colágeno tipo I murino povoado por fibroblastos humanos com queratinócitos humanos semeado na superfície (grupo estudo), formando um equivalente dermoepidérmico. Géis de colágeno povoado apenas por fibroblastos foram utilizados como grupo controle. Os critérios de confecção e armazenagem dos géis foram iguais para ambos os grupos. Resultados: Houve aumento evidente e estatisticamente significante na contração de gel das amostras povoadas por queratinócitos, em comparação ao grupo controle. Conclusões: Esses resultados sugerem que os queratinócitos não só podem modular a proliferação de fibroblastos, mas também, por si só, desempenhar papel ativo na contração da ferida. Novas investigações sobre mecanismos envolvidos nas vias de comunicação entre células e entre célula e matriz devem ser avaliadas sob o ponto de vista de participação dos queratinócitos na cicatrização de feridas e formação de cicatrizes patológicas.
Palavras-chave: Queratinócitos. Cicatrização. Técnicas de cultura de células. Fibroblastos.
Tissue damage induces a sequence of biochemical and cell reactions that are intended to repair the integrity of tissues. The healing process involves a tuned "orchestra" of cells, in which each participant interacts with others.
Many aspects of this intercellular communication remain unclear, despite the unquestionable fact that cells are responsible for growth factor release and other signaling molecules that actively participate in the wound healing process, modulating the microenvironment and cellular responses1,2. Among the components of this orchestra, fibroblasts perform many functions in wound healing. First, fibroblasts proliferate at the wound site and secrete collagen in the extracellular matrix, filling the void and creating a new structural support for the epidermis to proliferate. Second, fibroblasts contract the wound bed, an action that begins 4 to 5 days after injury and reaches its peak 12 to 15 days after injury. This feature can persist for longer periods, especially if the wound is still open3-7.
Bioengineering has revolutionized research in the healing area, enabling the establishment of in vitro models and cell culture systems that mimic different stages of wound healing. In particular, keratinocyte cultures on dermal substitutes provide an improved setting for observing these cells with the underlying matrix.
In 1979, Bell et al.8 established the model of a murine collagen gel populated with fibroblasts, whose contractile function determined by such cells could be assessed and modified by the action of modulators. This became a classic model for collagen remodeling assessment and wound contraction studies9,10.
The possible involvement of keratinocytes in wound remodeling was suggested by Souren et al.11 in 1989. These authors reported that collagen gels could be rearranged if keratinocytes were seeded on their surfaces, an effect that was not observed if the cells were seeded inside a gel.
Research on dermo-epidermal substitutes by Ralston et al.12 using a collagen gel populated with fibroblasts as a bed for cultured keratinocytes revealed that keratinocytes participate in the contraction of the extracellular matrix.
During the preparation of dermo-epidermal composites in the research laboratory where this study was conducted, it was observed that the epidermis had an area larger than its dermal substrate when it was released from the dermis, as if the keratinocyte layer had been released from the force of contraction and adhesion. It was also observed that dermal surfaces populated with keratinocytes suffered progressive contraction as the cells proliferated and reorganized in a confluent and multi-layered epithelium13. These intriguing observations reinforce the suggestion that keratinocytes play an active role in wound contraction.
This study aimed to assess and quantify the potential role of keratinocytes in wound contraction using an in vitro and preset model of collagen gel contraction populated with fibroblasts.
METHODS
Primary Culture of Keratinocytes and Fibroblasts
Human keratinocytes and fibroblasts were isolated from surgical remains of skin donated for research by five healthy women ranging in age from 18 to 42 years.
Keratinocytes were isolated by chemical enzymatic dissociation, as described by Rheinwald & Green14. Cells obtained between the second and fifth passages were counted and resuspended in culture medium containing 60% Dulbecco's Modified Eagle's Medium (DMEM) (GIBCO - Life Technologies, Baltimore, USA), 30% Ham 12% (GIBCO - Life Technologies, Baltimore, USA), 10% fetal calf serum (GIBCO - Life Technologies, Baltimore, USA), 4 mM glutamine (GIBCO - Life Technologies, Baltimore, USA), 0.18 mM adenine (Sigma Chemical - St. Louis, USA), 5 µg/ml insulin (Sigma Chemical - St. Louis, MO, USA), 0.4 µg/ml hydrocortisone (Sigma Chemical - St. Louis, USA), 0.1 mM cholera toxin (Sigma Chemical - St. Louis, USA), 2 mM triiodothyronine (Sigma Chemical - St. Louis, USA), and an antibiotic solution (penicillin 100 UI/ml/streptomycin 100 µg/ml) (Sigma Chemical - St. Louis, USA).
Primary keratinocyte cultures were established by seeding cells (5 × 106) suspended in 5 ml of the culture medium described above in 25-cm2 cell culture flasks (Falcon). Cells were maintained in an incubator (Fisher) with an atmosphere of 5% CO2 at 37 °C. After 24 hours, 10 µg/ml of epidermal growth factor was added to the culture medium (Sigma Chemical - Louis, USA). The medium was changed every 48 hours until the cells became subconfluent, after which they were trypsinized and seeded on collagen gels previously populated by fibroblasts.
Human fibroblasts were obtained by the explantation technique described by Carrel & Burrows15: skin fragments of partial thickness and devoid of subcutaneous tissue were attached to 25-cm2 surface culture plates and bathed in DMEM culture medium, 10% fetal calf serum, and 1% antibiotic solution (100 IU/ml of penicillin/streptomycin 100 µg/ml). The culture medium was changed every three days, and cells were kept in an incubator with an atmosphere of 5% CO2 at 37 °C. Fibroblasts migrated to the exterior of the skin fragments after a few days, and the fragments were subsequently removed while the cells proliferated on the flask's surface. After reaching subconfluency, cultures were repeatedly amplified until the desired number of cells was obtained.
Preparation of a Collagen Matrix Populated with Fibroblasts
The collagen solution was prepared according to the protocol proposed by Bell et al.8, in which the tail tendons of Wistar-Furth type rats were aseptically removed and placed in a container with diluted acetic acid at a ratio of 1:1000 (250 ml of acid solution/tail). The mixture was stored at 4 °C. After two weeks, a viscous solution was obtained that split into the following two phases after centrifugation at 500 rpm for one hour: a supernatant fraction that mainly contained solubilized type I collagen, which was removed and stored at 4 °C (soluble acid), and a residual fraction that represented acid-insoluble collagen, which was discarded.
The protocol proposed by Collagen Biomaterials16 was used to produce type I collagen gels. The gels consisted of a mixture of 100 µl of PBS (10×) with 100 µl of sodium hydroxide (NaOH) (0.1 M), 400 µl of the fibroblast suspension (5 × 104) in PBS (1×), and 400 µl of collagen solution.
Two-thirds of the prepared gels were used for keratinocyte seeding (hereafter called co-cultures, i.e., keratinocytes in a collagen gel populated with fibroblasts), and a third of these were used as control samples (collagen gels containing only keratinocytes).
Keratinocyte Seeding on Collagen Gels Containing Fibroblasts
After collagen gels containing fibroblasts had been polymerized for 12 hours, keratinocytes (1.2 × 105) were seeded on the surface of each gel. Both co-cultures and controls were embedded in the culture medium and kept in an incubator at 37 °C and 5% of CO2 for another 48 hours. This procedure was repeated in duplicate for each of the five different keratinocyte cell donors.
Collagen Gel Contraction
The area of the collagen gel populated with fibroblasts was photographed using a digital camera1 (SONY Cyber-shot, 3.2 megapixels) at 0, 12, 24, 36, and 48 hours after detachment from the well matrices. Gel area measurement was performed using the software UTHSCSA Image Tool for Windows version 3.017. The calculation was performed in triplicate and the resulting value represented the arithmetic mean of the triplicates, which was obtained in pixels and converted to square centimeters.
Statistical Analysis
The areas of the gels (co-culture and control) at the measured intervals were assessed using the nonparametric statistical test of ANOVA.
RESULTS
Progressive contraction of matrices occurred within a range of 48 hours after the gels were released from the walls of the wells. Macroscopically detectable contraction was not observed after this period (Figure 1).
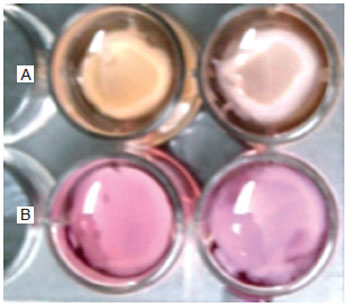
Figure 1 - Matrix contraction 48 hours after gels were released from the walls of the wells. A, a collagen gel populated with fibroblasts and keratinocytes (study group). B, a collagen matrix populated only with fibroblasts (control group).
Table 1 shows the average area of control gels (fibroblast/collagen gel) during different intervals (initial - time 0 = 1.96 cm2 area). The average areas of co-culture gels (keratinocytes + fibroblasts/collagen gel) measured at different intervals are shown in Table 2 (initial - time 0 = 1.96 cm2 area).
The contraction of control gels was significantly smaller (approximately 0.27 cm2 area reduction) compared to the contraction of co-culture gels (mean reduction of 1.53 cm2). Figure 2 shows the different contractions of collagen gels populated only by fibroblasts (controls) and keratinocytes seeded on collagen gels populated with fibroblasts (co-cultures) for the period of the experiment.
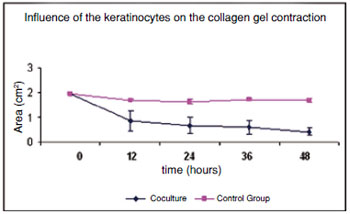
Figure 2 - Model of a collagen matrix populated with cells (cm2) in relation to the experimental time frame (P < 0.0001).
The gels seeded with keratinocytes (co-cultures) showed significantly greater contraction than the control group (P < 0.0001 and α = 0.05).
DISCUSSION
This experimental study assessed changes in the contraction of collagen gels populated with fibroblasts in the presence of keratinocytes.
The experimental model of extracellular matrix contraction has been used in wound healing studies since its development by Bell et al.8. Scattered fibroblasts in the collagen matrix behave more similarly to fibroblasts in vivo than those grown in a monolayer. Likewise, proliferation is slower in cells scattered in the extracellular matrix.
Generally speaking, three-dimensional models are best used in the study of relationships and interferences between components of the analyzed system, whereas monolayer cultures better assess behavior and cellular activity. However, some authors observed that the initial construction of the model may modify the results of gel contraction measurement18,19.
Bell et al.8 showed that after 48 hours of the onset of contraction, the matrix becomes stable without significant signs of collagen gel contraction. This information was used to establish the timetable proposed in this paper.
The literature indicates that gels attached to well walls exhibit reduced thickness without changes in the diameter. However, the mechanical release of gels from the walls results in a three-dimensional contraction of these gels, with thickness and diameter changes due to the random distribution of collagen fibers and fibroblast action over such fibers.
Another factor that may modify model contraction is the number of fibroblasts seeded in the collagen solution: in theory, the observed contraction increases as the number of cells increases. However, after a certain number of fibroblasts is reached per volume unit of collagen solution, no apparent significant increase or measurable change is observed in the matrix contraction19. The literature indicates that quantities between 3 × 105 and 5 × 105 fibroblasts/well of 1.96 cm2 of area are sufficient to promote gel contraction consistent with what occurs in vivo20.
A previous studies conducted in the same laboratory as the work described herein determined that less than 5 × 104 fibroblasts seeded in 1.96 cm2 wells do not generate contraction (unpublished data). Therefore, this number of cells was selected for the present study so that there was less influence of fibroblasts in the contraction of these gels, thus allowing better characterization of the participation of keratinocytes in this phenomenon.
These results clearly demonstrate that the collagen gels populated with fibroblasts that received keratinocytes had significantly higher rates of contraction compared to controls. The final area of gels seeded with keratinocytes was up to five times smaller than the area of control gels. Given that the systems were similar and varied only in the action of keratinocytes, it is clear that keratinocytes have the ability to interfere with the function of collagen substrate reorganization of fibroblasts.
Data from this study suggest that several concepts regarding the contractile and proliferative phases of wound healing should be revised. Although most experts believe that fibroblasts are solely responsible for wound contraction and collagen rearrangement, the present study suggests a clear collaboration of keratinocytes in these processes. However, the exact mechanisms underlying this participation must be better understood.
CONCLUSION
Keratinocytes play a role in extracellular matrix reorganization. The experimental model of contraction used in this paper may allow further investigation of the communication pathways between interacting cells and modulators, providing new insight into the role of keratinocytes in wound healing and pathologic scarring.
REFERENCES
1. Peacock EE, Cohen IK. Wound healing. In: McCarthy J, ed. Plastic surgery. Philadelphia: WB Saunders; 1990. p. 161-85.
2. Grinnel F. Fibroblasts, myofibroblasts, and wound contraction. J Cell Biol. 1994;124(4):401-4.
3. Finesmith TH, Broadley KN, Davidson JM. Fibroblasts from wounds of different stages of repair vary in their ability to contract a collagen gel in response to growth factors. J Cell Physiol. 1990;144(1):99-107.
4. Coulomb B, Lebreton C, Dubertret L. Influence of human dermal fibroblasts on epidermalization. J Invest Dermatol. 1989;92(1):122-5.
5. Gabbiani G, Ryan GB, Majne G. Presence of modified fibroblasts in granulation tissue and their possible role in wound contraction. Experientia. 1971;27(5):549-50.
6. Darby I, Skalli O, Gabbiani G. Alpha-smooth muscle actin is transiently expressed by myofibroblasts during experimental wound healing. Lab Invest. 1990;63(1):21-9.
7. Ross R. The fibroblast and wound repair. Biol Rev Camb Philos Soc. 1968;43(1):51-96.
8. Bell E, Ivarsson B, Merrill C. Production of tissue-like structure by contraction of collagen lattices by human fibroblasts of different proliferative potential in vitro. Proc Natl Acad Sci USA. 1979;76(3):1274-8.
9. Kamamoto F, Paggiaro AO, Rodas A, Herson MR, Mathor MB, Ferreira MC. A wound contraction experimental model for studying keloids and wound-healing modulators. Artif Organs. 2003;27(8):701-5.
10. Isaac C, Mathor MB, Bariani G, Paggiaro AO, Herson MR, Goldenstein-Schainberg C, et al. Pentoxifylline modifies three-dimensional collagen lattice model contraction and expression of collagen types I and III by human fibroblasts derived from post-burn hypertrophic scars and from normal skin. Burns. 2009;35(5):701-6.
11. Souren JM, Ponec M, van Wijk R. Contraction of collagen by human fibroblasts and keratinocytes. In Vitro Cell Dev Biol. 1989;25(11):1039-45.
12. Ralston DR, Layton C, Dalley AJ, Boyce SG, Freedlander E, MacNeil S. Keratinocytes contract human dermal extracellular matrix and reduce soluble fibronectin production by fibroblasts in a skin composite model. Br J Plast Surg. 1997;50(6):408-15.
13. Herson MR, Mathor MB, Altran S, Capellozzi VL, Ferreira MC. In vitro construction of a potential skin substitute through direct human keratinocyte plating onto decellularized glycerol-preserved allodermis. Artif Organs. 2001;25(11):901-6.
14. Rheinwald JG, Green H. Serial cultivation of strains of human epidermal keratinocytes: the formation of keratinizing colonies from single cells. Cell. 1975;6(3):331-43.
15. Carrel A, Burrows MT. Cultivation of adult tissues and organs outside the body. JAMA. 1910;55:1379-81.
16. Collagen biomaterials. Available at: http://www.vasoseal.com/cb/cbbiomaterials.html. Access on: 5/2/2011.
17. Wilcox CD, Dove SB, McDavid WD, Greer DB. UTHSCSA ImageTool, Version 3.0 Final. Available at: http://ddsdx.uthscsa.edu/dig/itdesc.html
18. Rompré P, Auger FA, Germain L, Bouvard V, López Valle CA, Thibault J, et al. Influence of initial collagen and cellular concentrations on the final surface area of dermal and skin equivalents: a Box-Behnken analysis. In Vitro Cell Dev Biol. 1990;26(10):983-90.
19. Zhu YK, Umino T, Liu XD, Wang HJ, Romberger DJ, Spurzem JR, et al. Contraction of fibroblast-containing gels: initial collagen concentration regulates the degree of contraction and cell survival. In Vitro Cell Dev Biol Anim. 2001;37(1):10-6.
20. Dallon JC, Ehrlich HP. A review of fibroblast-populated collagen lattices. Wound Repair Regen. 2008;16(4):472-9.
1. Doctor, head of the Research Laboratory of Cell Culture and Wound Healing (LIM 04) of the Department of Plastic Surgery of Hospital das Clínicas of the School of Medicine of Universidade de São Paulo (HCFMUSP), São Paulo, SP, Brazil.
2. Doctor, head of the Tissue Bank of the Central Institute of HCFMUSP, São Paulo, SP, Brazil.
3. Practitioner Resident of Plastic Surgery at HCFMUSP, São Paulo, Brazil.
4. Doctor, assistant professor at Monash University, Australia
5. MSc, researcher at the Laboratory of Cell Culture of the Department of Plastic Surgery of HCFMUSP, São Paulo, SP, Brazil.
6. Post-doctoral degree, researcher at the Institute of Energy and Nuclear Research (IPEN), São Paulo, SP, Brazil.
7. Full Professor at the Discipline of Plastic Surgery of HCFMUSP, São Paulo, SP, Brazil.
Correspondence to:
César Isaac
Av. Doutor Arnaldo, 455 - sala 1.360 - Pinheiros
São Paulo, SP, Brazil - CEP 01246-903
E-mail: cesaris@uol.com.br
Submitted to SGP (Sistema de Gestão de Publicações/Manager Publications System) of RBCP (Revista Brasileira de Cirurgia Plástica/Brazilian Journal of Plastic Surgery).
Received: June 7, 2011
Accepted: July 12, 2011
Study conducted at the Research Laboratory of Cell Culture and Wound Healing (LIM 04) of the Department of Plastic Surgery of Hospital das Clínicas of the School of Medicine of Universidade de São Paulo (HCFMUSP), São Paulo, SP, Brazil.